Researchers from ice2ice Physics of Ice, Climate and Earth (PICE) at the Niels Bohr Institute, University of Copenhagen, have succeeded in making a method to enlighten an otherwise dark period in climate history. Working with the ice core ReCap, drilled close to the coast in East Greenland, postdoc Marius Simonsen wondered why the dust particles from the interglacial period – the warmer period of time between the ice ages – were several times bigger than the dust particles from the ice age. His research led to the invention of a method able to map the advancement of the glaciers in cold periods and the melting in warmer periods. This data is important for the climate models we use to predict sea level rise. The result is now published in Nature Communications.
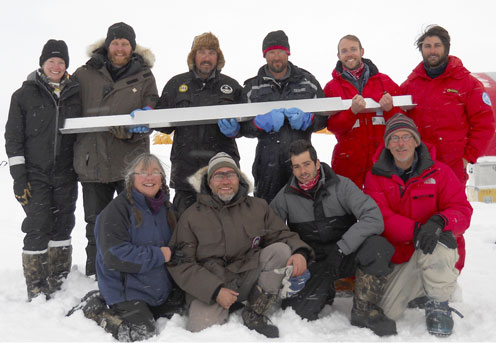
The larger particles of dust don’t travel far – they come from East Greenland
Based on the hypothesis that the bigger dust particles in the ice couldn’t have come from afar, the then PhD student, Marius Simonsen, examined the dust at select locations on the coast of East Greenland close to the drill site. The chemical composition turned out to be similar to the larger particles in the ice. The smaller particles of dust caught in the ice, on the other hand, travel from Asia, blown to Greenland by dust storms. In other words, the bigger particles of dust in the ice must mean presence of bare land close to the drill site. The ice is composed of annual layers, like the growth rings in a tree, so the distribution of large and small dust particles can be linked to the advancement and melting of the ice. Large particles mean bare land in the vicinity, small particles mean the land is covered in ice. The end of the ice age, where the ice cap is receding, is well explained scientifically, Marius Simonsen explains. “But it is very difficult to retrieve data on the period before the ice age. The ice is an extremely strong natural force, so it grinds away everything when advancing. But with the new method, we have data on the advancement of the ice. All of a sudden, we have a link to information on how quickly we enter an ice age, in a way we never used to have”.
Gaining knowledge on Glacier reaction to atmospheric content of CO2 is crucial
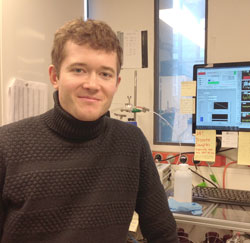
It is important to know more about how glaciers react to changes in the atmosphere, and rather a lot is known on the composition of the atmosphere during the ice age. The results from the new method can now be used to compare the reaction in the masses of ice to changes in the atmospheric content of greenhouse gasses like CO2. Marius Simonsen says: “The glaciers receded at the beginning of the present interglacial, just like they do today because of climate change. The two scenarios are not entirely comparable, because there was much more inland ice then than now by the coast in East Greenland where the ice core is drilled. Never the less, the results are very interesting indeed when making climate models, because the models must be tested by comparison to reality. And in the new method we’ve acquired an anchor point in a period about which not much scientific knowledge existed”. The method helps putting constraints on our knowledge on the influence of greenhouse gasses on ice melting and, consequently, sea level.
The method can provide us with new information on how fast the glaciers recede
Helle Astrid Kjær, Assistant professor in PICE, says the objective of PICE now is to utilize the new method at other locations so we can gather more data on the changes of glaciers in the past. The researchers are already planning new drill sites in North East Greenland and Canada. It is very likely that advancement and melting of the ice there is different from East Greenland. “Perhaps, with the new method, we are able to see how fast the ice age came in from the north and moved south”, Helle Astrid Kjær says.
It is a precondition for the feasibility of the method that bare land exists in the vicinity of the drill site, so dust particles can be found. This was the case during the last interglacial as the temperature then, app. 115,000 years ago, was up to 8 degrees C. warmer than today, according to a former study from the Niels Bohr Institute. Hence, the method will most likely be usable in North East Greenland and Canada. The researchers at the Niels Bohr Institute are already establishing new collaborations with Canadian researchers based on the new method.
Drilling of the ice core ReCap was supported by The Danish National Research Foundation, the American National Science Foundation, the German Alfred Wegener Institute and the European Union Horizon 2020 Research and Innovation Programme. The measurements of dust were supported by the EU funding ice2ice and Horizon 2020 – TIPES